Exploring the “C’s”: Climate Change and Cold-water Corals
Jay Lunden
Temple University
With our society's current focus on sustainability and global climate change, it’s hard for most people to avoid or ignore this topic of concern for our planet. However, many people may not realize that climate change is extending its nasty tendrils to the ocean depths, negatively impacting many of its most notable inhabitants: cold-water corals. These cold-water corals, such as Lophelia pertusa, are able to tolerate only a narrow range of temperature levels. As our planet is warming, seawater temperatures are also changing. Furthermore, a coral’s ability to lay down its skeleton is also being affected by increasing carbon dioxide levels in our planet’s atmosphere. Let's explore these two phenomena and look at what the researchers on Lophelia II cruise are doing to learn more about it.
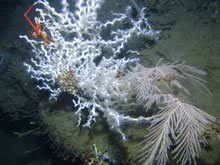
Colonies of Lophelia pertusa (left) and Callogorgia americana (right) provide habitat for various invertebrates, such as galatheid crabs (on L. pertusa) and brittle stars (on C. americana). Click image for larger view and image credit.
Lophelia pertusa polyps extend from the corallites (the skeleton of the polyp) in order to feed. Click image for larger view and image credit.
Let’s take a look at ocean warming and its potential impacts on cold-water corals. The highest fluctuation in seawater temperature levels occurs at the surface, due to its position in the water column. However, seawater is not static; it moves via currents, upwelling, and downwelling, which are mass movements of water up or down the water column. These can result in temperature changes even at great depths, such as those where cold-water corals are found. Furthermore, seasonal fluctuations have been reported at sites containing cold-water corals. With an incremental change of 3°C (37ºF), researchers observed a tripling of the metabolic rate of Lophelia pertusa. When metabolic rate increases, an animal will require an equivalent increase in food intake to compensate for such a change. However, recall that cold-water corals lack photosynthetic symbionts and are therefore unable to harvest the sun’s energy to meet their metabolic requirements. Therefore, any food intake compensation is dependent on how much food the coral polyp can capture from the water column, which is dependent on primary production at the surface.
How will corals compensate for this potential lack of food? We don’t know. Furthermore, how will mortality of corals be impacted with rising seawater temperatures? That is one question we hope to answer during the course of this project.
As if rising seawater temperatures was not enough of a problem for cold-water corals, we also have to investigate rising atmospheric carbon dioxide levels and its effect on oceanic carbonate chemistry. Carbon dioxide (CO2) is a byproduct of industrial processes, the burning of fossil fuels, and even our own respiration. Atmospheric CO2 has increased steadily since the Industrial Revolution; and approximately 20% of this “anthropogenic” (human-produced) CO2 has been absorbed by the oceans. When CO2 interacts with water molecules (H2O), atoms from each molecule are exchanged with each other and we observe the following equilibrium:
H2O + CO2 <-> H2CO3 <-> HCO3- + H+ <-> CO32- + 2H+
Two of the most important components of this system to cold-water corals are the HCO3- (bicarbonate) and CO32- (carbonate) molecules. Cold-water corals, as well as their shallow-water relatives, make their skeletons out of calcium carbonate (CaCO3), which is essentially limestone. However, with more CO2 dissolving into seawater, the levels of bicarbonate (HCO3-) and carbonate (CO32-) decrease as more H+ ions are released from H2O molecules. This results in a less favorable environment for coral calcification to occur, and is known as “ocean acidification.”
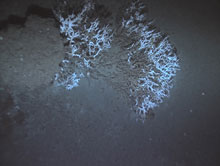
A top-down view of Lophelia pertusa colonies at Viosca Knoll 826. Click image for larger view and image credit.
Shoaling of the aragonite saturation (ASH) horizon poses a significant threat to calcifying organisms in deep water. Click image for larger view and image credit.
One of the metrics marine biologists use to assess the carbonate chemistry of a particular area of the ocean is called the aragonite saturation state. Aragonite is the form of calcium carbonate (CaCO3) that stony corals, such as Lophelia pertusa and Madrepora oculata, use to lay down their skeletons. We will be measuring the aragonite saturation state of several coral sites during this expedition. This information will give us an idea of the relative availability of bicarbonate and carbonate to the corals at our sites. Furthermore, it will allow us to predict how well these areas can resist changes in oceanic pH, and therefore, ocean acidification.