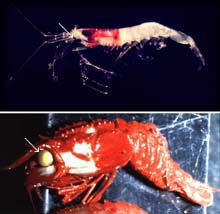
Note the difference in the eye size (white arrows) of the pelagic species Systellaspis (above) that lives between 500-700 m depth, and Glyphocrangon (below) that lives between 700-1500 m depth. Click image for larger view.
Visual Ecology and Bioluminescence
Tamara Frank
Biological Oceanographer, Harbor Branch Oceanographic Institution
Edith A. Widder
Senior Scientist, Harbor Branch Oceanographic Institution
Many deep-sea benthic animals have very large eyes, but the sensitivity level of these eyes, as well as what they are used for, has remained a mystery. The issue is confounded further by the fact that the eyes of pelagic animalscreatures that live in the water columndecrease in size after a certain depth, as sunlight penetration dims. Why then, do benthic species have large eyes at depths where pelagic species have small eyes?
The answer likely lies in the phenomenon known as bioluminescencelight generated by plants or animals. One familiar source of bioluminescence is the common firefly. However, bioluminescence is much more common in the ocean than on land. In fact, an estimated 90 percent of the animals that live below 500 m in the oceans pelagic zone are bioluminescent. Common bioluminescent animals in the pelagic zone include fish, squid, shrimp and jellyfish.
Scientists hypothesize that the huge eyes of deep-sea benthic creatures are adapted for viewing bioluminescence. But little is known about bioluminescence on the deep-sea floor, and we know virtually nothing about the visual systems of these deep-sea inhabitants.
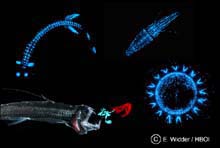
A common source of bioluminescence in the pelagic zone include fish, squid, shrimp and jellyfish. Click image for larger view.
Detecting Bioluminescence
To explore for new sources of bioluminescence in the benthic environment, we will use an underwater intensified silicon intensified target (ISIT) video camera mounted on the deep-sea submersible Johnson-Sea-Link (JSL). We also will be using an ultraviolet light to detect fluorescence, which can be, but is not always, an indicator of bioluminescence. If we discover any new sources of bioluminescence, we will collect them for shipboard studies, such as measurement of the color of the light, known as the emission spectra.
Additionally, we will be using a new camera system developed for ocean exploration called Eye-in-the-Sea. This deep-sea observation tool can be left on the sea bottom to record animal behaviors after the noisy, brightly-lit submersible leaves the vicinity. The system uses an especially sensitive camera that can record bioluminescence. The camera is activated by a light sensor that detects bioluminescent flashes. After the camera comes on and records some of the bioluminescence, a red light turns on, revealing the animal. Based on Dr. Franks recordings from the eyes of deep-sea animals, we believe this light should be invisible to the animals. We hope that Eye-in-the-Sea will reveal behaviors and possibly even animals that no one has ever seen before.
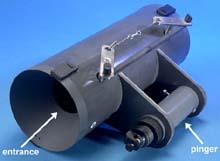
These specially designed traps isolate the shrimps and crabs inside from light and elevated water temperatures as they are brought to the surface. Click image for larger view.
Collecting Benthic Animals
Until recently, we have been unable to study the visual systems of these deep-sea species. Because their eyes are so sensitive to light, exposure to surface lights, even lights at night, permanently blinds them. Thus, standard commercial traps and trawls cannot be used to collect these animals. Instead, we will rely on special benthic traps designed by engineers at Harbor Branch Oceanographic Institute (HBOI) to collect specimens.
These traps are constructed from polyvinyl chloride (PVC) and are designed with funnels at the entrances. The funnels prevent a shrimp or small crab from escaping once it has entered the trap. Once the trap has been open for eight to 24 hours, the doors will close, leaving a trap that is light-tight and water-tight. A pinger attached to each trap emits a signal that allows the JSL to find and retrieve the trap. Because the traps are now closed, they can be picked up by the JSL without exposing the animals inside to the submersibles bright lights.
The animals inside also will be immersed in cold water (4 degrees C) throughout their ride back to the surface, which will prevent any rapid pressure and water temperature changes that often kill most deep-sea animals brought to the surface. Deep-sea shrimp, if they are kept in the cold, dark water to which their bodies are adapted, can survive for weeks on the surface.
Once the animals are brought to the surface, the traps will be carried to an environmentally controlled room kept at 4 degrees C, where they will be opened under dim red light. The captured specimens will be placed in light-tight, water-filled tanks until we are ready to work on them.
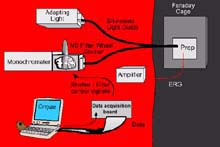
A schematic drawing of the electrophysiology apparatus used to study the color and light sensitivity of deep sea benthic organisms. Click image for larger view.
Electrophysiology
To study the eyes of the deep-sea crustaceans that we collect, we will remove their eyes and prepare them for structural studies, all under a dim red light. Using both transmission and scanning electron microscopy, we will observe whether there are any unusual optical adaptations that significantly increase the specimens sensitivity to light. Other studies have discovered a remarkable variety of unusual adaptations in the eyes of deep-sea organisms, such as biological light guides, scanning binoculars, huge parabolic mirrors, and vast arrays of reflecting mirrors behind the retina.
During this expedition, we also will be conducting physiological studies to determine what colors these deep-sea creatures see, and how sensitive their eyes are compared to shallow-water species. To do this, we must use an electrophysiological apparatus.
The crustacean being tested will be placed in a holder that allows its pleopods (legs on a shrimp) or gill bailers (on a crab) to move and generate respiratory currents across its gills. It is kept in a light-tight room, in a bath of chilled water, with an electrode on one eye. When the eye sees a flash of light, it converts the light energy into electrical energy, which is picked up by the electrode on the eye. This electrical signal is amplified and then stored as a digitized signal on the computer. With this set-up, we can flash lights of different colors on the eye using a device called a monochromator, which splits white light into its component colors. We simply dial in the color we want to use.
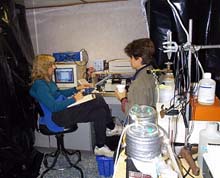
The actual electrophysiology laboratory setup on board ship. Black plastic and duct tape are often used to create as much of a light-free environment as possible. Click image for larger view.
The neutral density filter wheel on the front of the monochromator allows us to control the intensity of the light. The Faraday cage blocks out any ambient electrical noise. To transmit the light from the monochromator (and not the room) to the eye, we use a light guide. One end of the light guide is sealed to the monochromator. The other end goes through a little hole in the Faraday cage and transmits the light directly to the crustaceans eye. Based on the eyes response to the flash of light, we can determine what colors the crustacean sees best. For example, if the animal is more sensitive to blue light than red light, the eyes response to blue light will be much bigger than the response to red light of the same intensity.
We also may conduct experiments that will determine the critical flicker fusion (CFF) frequency in the crustacean. The CFF is the frequency at which a flickering light source is considered continuous. For example, todays movies are actually individual snapshots presented so rapidly that the flicker frequency is undetectable by humans. The rate is 24 frames per second. But each frame is flashed three times, thus increasing the flicker rate to 72 flashes per second, which is above our CFF. Hence, the motion of people walking looks fluid and continuous. Early movies, however, showed 16 frames per second, which is considerably slower than the human flicker fusion frequency, allowing us to detect the flicker between frames. Hence, the motion of people walking in these early movies looks halting and spastic. Though a crustacean is not interested in watching movies, it does need to be able to track its prey, and the CFF is an indication of how well it is able to do that.
Sign up for the Ocean Explorer E-mail Update List.